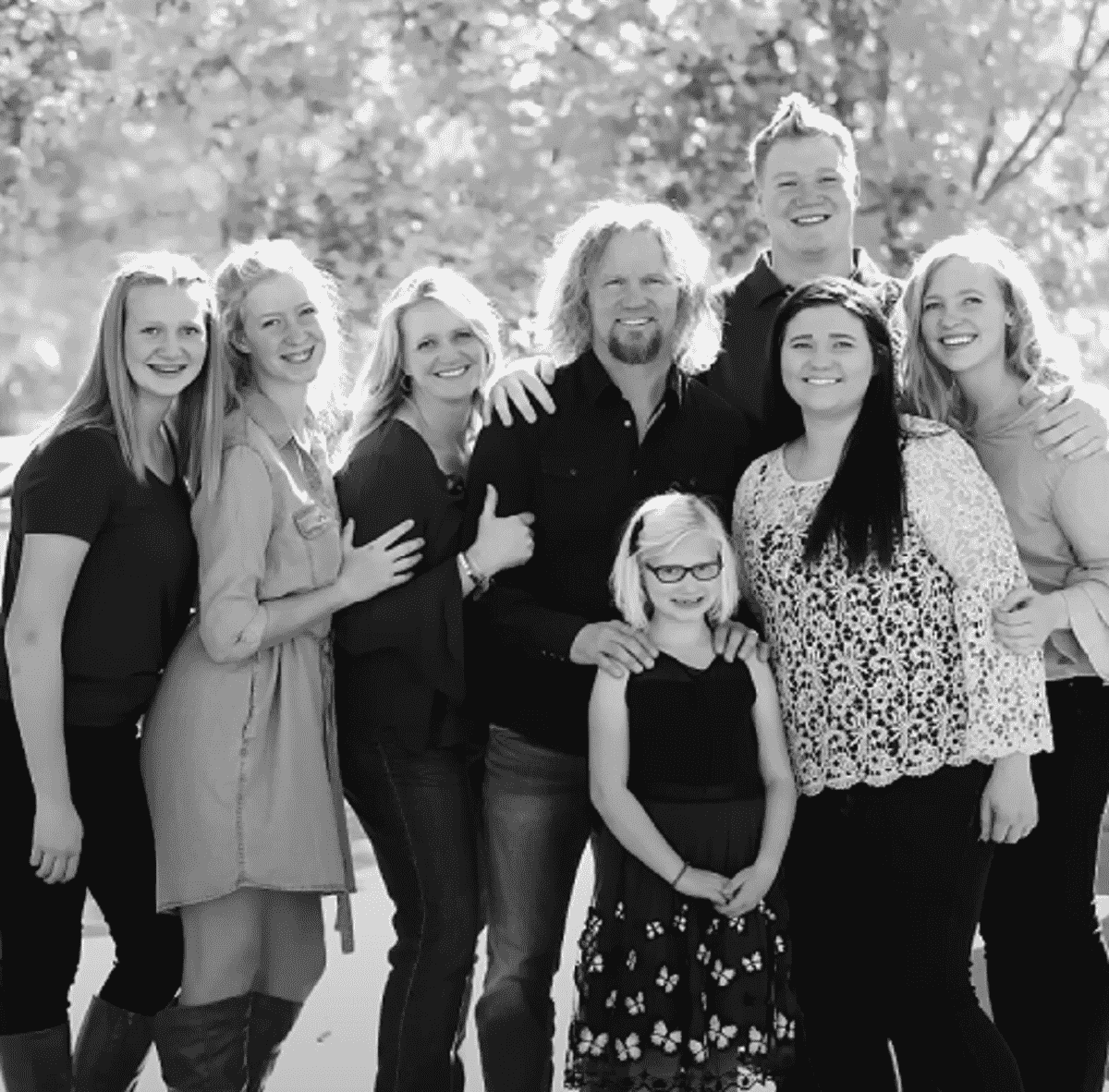
‘Sister Wives’ Drama: Paedon Might Not Be the Only Brown Kid Not on Board With Christine’s Whirlwind Romance
Christine Brown’s whirlwind romance has taken center stage in recent months. The Sister Wives star shocked fans when she announced her engagement to David Woolley just weeks after revealing she was in a relationship with the Utah-based contractor. While fans and most of her family seem happy about the quick development of her love life, her son, Paedon Brown, decidedly is not. Recent social media posts have some fans thinking there is another Brown child who is less than enthralled with how quickly Christine Brown moved on. Truely Brown, Christine’s youngest child, doesn’t look completely comfortable in recent social media posts, theorize followers.
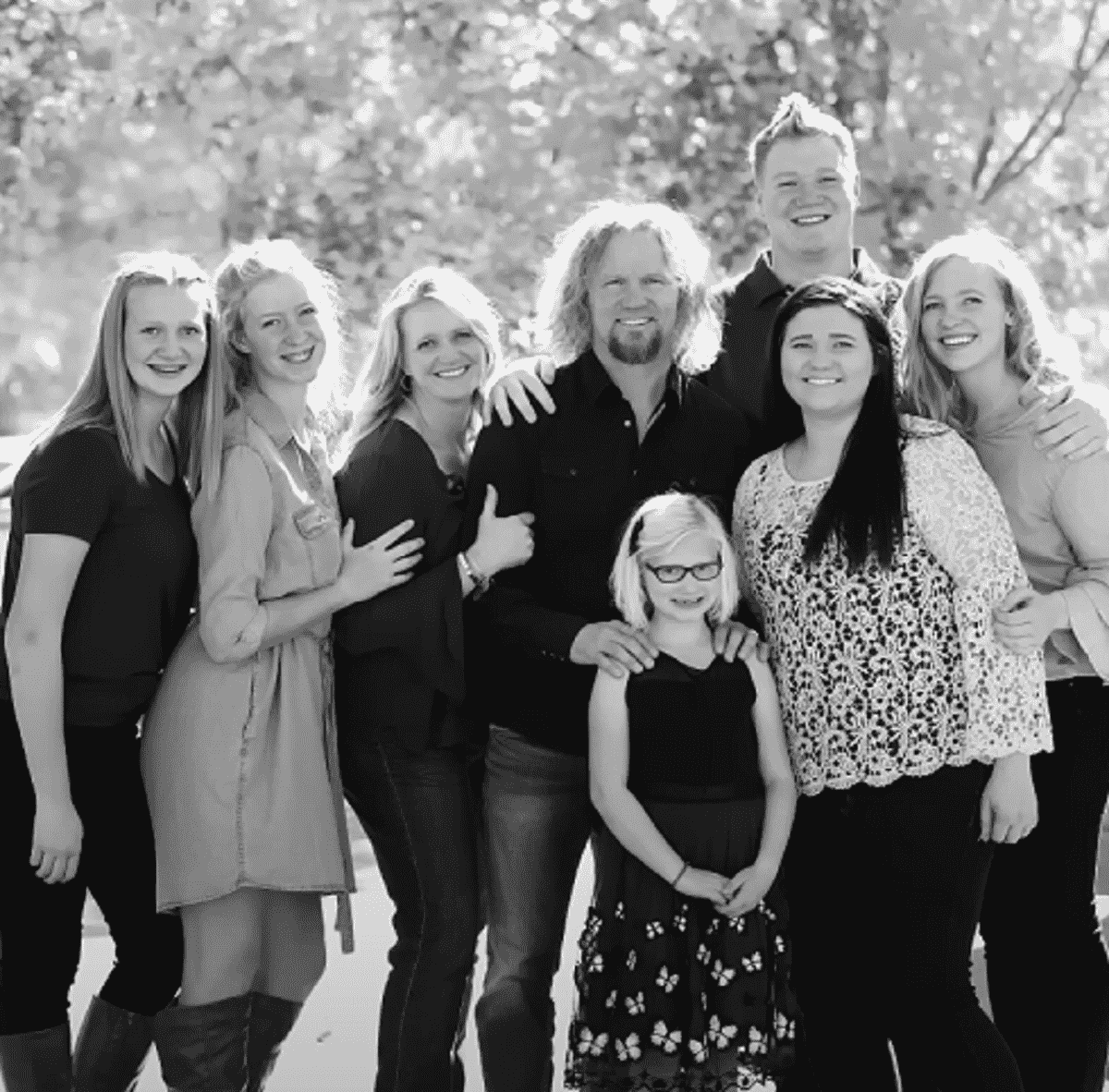
Recent photos have ‘Sister Wives’ fans questioning Truely Brown’s feelings about Christine Brown’s new relationship
Christine Brown’s social media followers worry that Truely Brown isn’t comfortable with her mom’s new romance following a series of new photos posted to Christine’s Instagram account. Christine Brown and her new love took a trip to California with a couple of her kids. Ysabel Brown seems to be completely comfortable around David Woolley, based on the pictures. Fans have concerns for Truely, though. Several social media followers pointed out that Christine’s youngest child doesn’t appear particularly thrilled. Truely Brown’s body language seems to indicate that she’s hesitant to embrace the new man in her mom’s life.
In Christine’s photos, Truely is seen ignoring David in one, reading a book while he smiles for the camera. Another image, a group hug shot, had weird vibes, too, argued fans. Fans quickly noticed that Truely seemed to be pulling away and protecting her space. In another image, Truely appears to be trying to get closer to her sister while moving away from David. Several fans commented on the 13-year-old’s odd body language. They wonder if Truely is having difficulty adjusting to yet another big change in her young life. Other commenters insist everything looks fine.
Paedon Brown has made his feelings about his mom’s romance known
Truely doesn’t have social media and has never commented publicly on her parents’ divorce or her mom’s new man. Her brother, however, does. Paedon Brown has been more than happy to talk. Paedon, Christine and Kody’s only son together, has taken to TikTok to discuss his mother’s love life more than once.
The TikToker noted that he believed his mother moved too quickly with David, especially since she had only gotten out of a bad marriage a year earlier. He alleges he had a conversation with his mother about it, too. The sentiment doesn’t seem to be shared by most of his siblings, but the Sister Wives fan base has debated the quick engagement since the news broke.
Things are progressing regardless of how Paedon Brown, Truely Brown, or the Sister Wives community feel about Christine Brown’s romance. Christine has yet to share her wedding date with fans, but she did take to Instagram to ask for vendor recommendations. While we don’t know when it will happen, the nuptials seem imminent. The couple recently purchased their first home together.