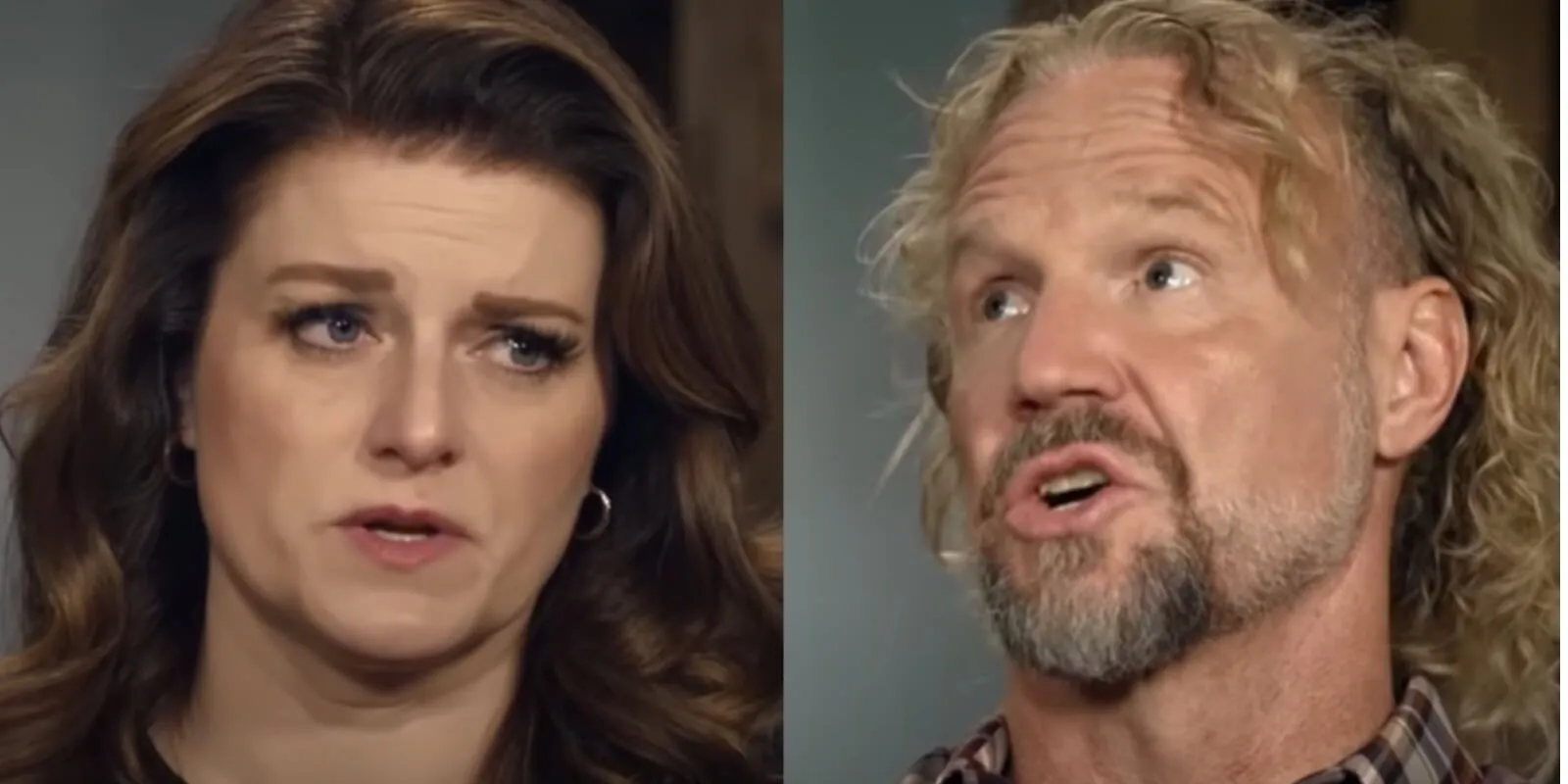
‘Sister Wives’: 3 Ways Robyn Brown Made the Ultimate Fool of Kody Brown
Kody Brown has been at the heart of his family’s collapse during 19 seasons of Sister Wives. TLC led viewers to believe Kody made the decisions that caused his polygamist family to unravel. However, Robyn Brown, his fourth wife, played a much bigger role—not only in the family’s downfall but reportedly, in making a fool of Kody.
3. Robyn manipulated the instability of the Brown family to her advantage
Throughout her and Kody Brown’s marriage, Robyn Brown has been accused of manipulating the Brown family. As his fourth wife, Robyn appeared to notice the weak spots in his relationships with Meri, Janelle, and Christine Brown and use them to her advantage.
Early in their relationship, Robyn quickly became Kody’s favorite wife. Thus, this shift in Kody’s attention created tension within the family.
Robyn’s strategic approach to securing Kody’s favor made it clear she had a significant influence over him. Therefore, Kody began prioritizing her needs and desires, causing friction with his other wives who felt overlooked.
Perhaps her biggest manipulation was Kody’s relationships with Meri and Janelle. Robyn wanted Kody to remain in loveless marriages with them for selfish reasons. Therefore, she appeared to play to the trio’s weaknesses to guilt them into remaining married when they were better apart.
2. Creating family discontent by playing ‘victim’
Robyn frequently portrays herself as the Brown family’s victim. This leads Kody to side with her and play a protective role against anyone whom he feels disrespects her.
Whether it was her struggles with the other wives or her overall emotional distress, Robyn often put herself at the center of the narrative, positioning Kody as the one who had to fix her problems. But Kody was disrespected for his behavior against his family in favor of Robyn, which made him appear a fool.
By constantly playing the victim, Robyn ensured that Kody would focus his efforts on her happiness. This led to the family’s downfall and made Kody appear uncaring to his other wives and their shared children.
1. Kody’s blind spot for Robyn’s manipulation
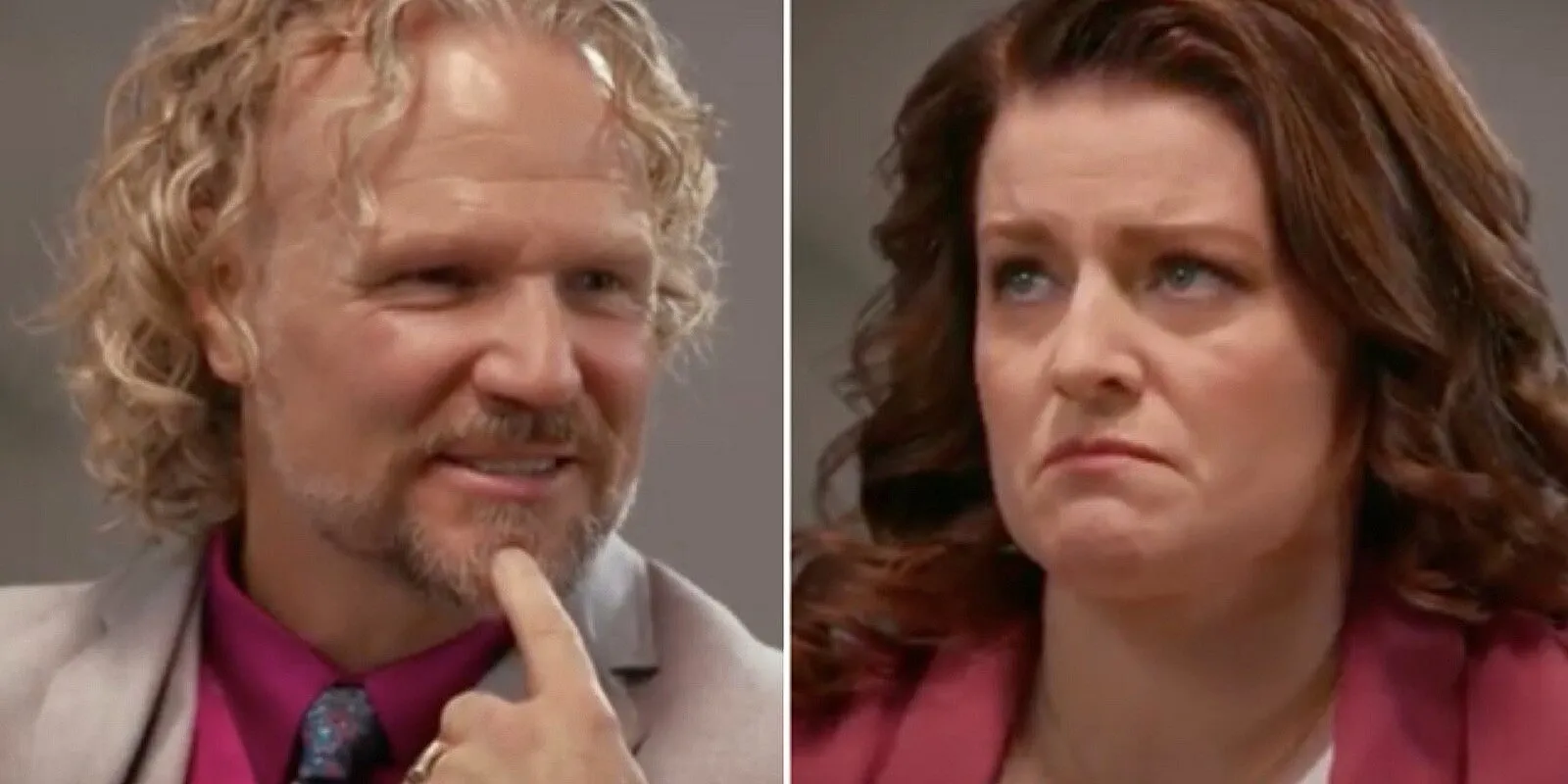
Kody Brown’s inability to see through Robyn Brown’s manipulation is exactly why she’s been able to fool him. Thus, once a confident leader who captivated his wives, he’s now the punchline in his remaining relationship.
Additionally, Robyn’s ability to have Kody wrapped around her finger created a situation in which Kody was unable to see how his decisions were being shaped by her influence rather than his own judgment.
Most recently, Sister Wives fans learned that while Kody maintained he didn’t have enough funds to buy out Janelle and Meri Brown for their part of the family’s shared Coyote Pass property, he did have enough to put down $420,000 on a new 7,884-square-foot home for Robyn and their children, according to In Touch. The couple reportedly carries a loan of $1,680,000 as a mortgage.
The couple’s excessive spending has been reported on social media for years. Jewelry, paintings, home decor, expensive ATVs, and other items were commented on by Janelle Brown, during a season 19 episode of Sister Wives.
But the most embarrassing way Robyn Brown continues to make a fool of Kody is by recklessly spending their money. Social media has been abuzz over reports she’s spent tens of thousands of dollars on Etsy. The end of her URL features her maiden name, Sullivan, and the page shows all 235 of her favorite stores, including multiple doll retailers.
Sister Wives is currently on hiatus. The show returns to TLC in the spring.